Factors Affecting Vibrational Frequencies: Infrared (IR) spectroscopy is a powerful analytical technique widely used to study the vibrational properties of molecules, helping scientists gain insight into molecular structures, functional groups, and bonding characteristics. The vibrational frequencies observed in IR spectroscopy are influenced by various intrinsic and extrinsic factors that determine the absorption positions of molecular vibrations. A detailed understanding of these factors is essential for accurate spectral interpretation and chemical characterization. Below are the key factors that affect vibrational frequencies in IR spectroscopy:
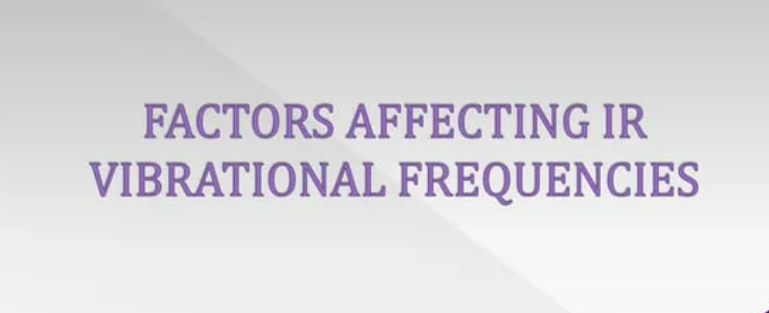
Factors Affecting Vibrational Frequencies
1. Mass of Atoms
The mass of the atoms involved in a vibration has a direct effect on the vibrational frequency of a bond. According to Hooke’s Law, vibrational frequency is inversely proportional to the square root of the atomic masses involved in the vibration. Lighter atoms vibrate at higher frequencies compared to heavier atoms for the same type of bond. For example:
- The C-H stretching vibration in methane (CH₄) appears at a higher frequency than the C-D stretching vibration in deuterated methane (CD₄). The heavier deuterium isotope lowers the vibrational frequency due to its greater mass.
- Similarly, O-H stretching vibrations occur at a higher frequency than S-H stretching vibrations because oxygen is lighter than sulfur.
2. Force Constant (Bond Strength)
The force constant (k) of a chemical bond is a measure of bond strength, and it significantly affects vibrational frequency. Stronger bonds with higher force constants exhibit higher vibrational frequencies, whereas weaker bonds with lower force constants show lower vibrational frequencies. This trend can be observed in different types of bonds:
- Triple bonds (C≡C, N≡N, C≡N) have the highest vibrational frequencies due to their high bond strength.
- Double bonds (C=C, C=O, C=N) have intermediate vibrational frequencies.
- Single bonds (C-C, C-N, C-O) exhibit the lowest vibrational frequencies. For example, the stretching frequency of the C≡C bond (~2100-2250 cm⁻¹) is higher than that of the C=C bond (~1600-1700 cm⁻¹) and much higher than the C-C bond (~1000-1200 cm⁻¹).
3. Bond Length
The length of a chemical bond affects its vibrational frequency, with shorter bonds typically having higher vibrational frequencies. Bond length and force constant are related, as shorter bonds usually indicate stronger interactions between atoms. Consequently:
- O-H bonds in alcohols and acids, being shorter and stronger, have high stretching frequencies (~3200-3600 cm⁻¹).
- C-H bonds have higher vibrational frequencies than C-C bonds due to shorter bond lengths.
4. Bond Angle
The bond angle in polyatomic molecules affects vibrational modes, especially bending vibrations. Changes in bond angles can alter force constants, affecting vibrational frequencies. Molecules with smaller bond angles may experience different vibrational characteristics compared to molecules with larger bond angles.
- The bending vibration in water (H₂O), where the H-O-H bond angle is ~104.5°, differs from that in carbon dioxide (CO₂), which has a linear bond angle (180°).
- Vibrational modes in cyclic compounds are also influenced by ring strain, affecting their IR absorption.
5. Dipole Moment Change
For a vibration to be IR active, it must result in a change in the molecule’s dipole moment. The greater the dipole moment change during a vibration, the stronger the IR absorption band.
- Highly polar bonds (C=O, O-H, N-H) exhibit strong absorption bands because their vibrations cause significant dipole moment changes.
- Nonpolar symmetric bonds (O₂, N₂, Cl₂) do not show IR absorption because their vibrations do not result in a dipole moment change, making them IR inactive.
6. Molecular Environment
The molecular environment of a functional group affects its vibrational frequency due to intermolecular interactions such as hydrogen bonding and van der Waals forces. These interactions can shift absorption frequencies and influence peak intensities.
- Hydrogen bonding lowers vibrational frequencies by weakening bonds. For example, the O-H stretching frequency in alcohols appears around 3600 cm⁻¹ in the gas phase but shifts to lower frequencies (~3200-3500 cm⁻¹) in hydrogen-bonded states.
- Solvent effects alter vibrational frequencies by stabilizing or destabilizing specific molecular conformations, leading to peak shifts.
- Steric hindrance from bulky substituents can alter bond strengths and vibrational frequencies.
7. Isotopic Substitution
Replacing an atom with its heavier isotope affects vibrational frequencies by decreasing the frequency due to increased atomic mass. This effect follows Hooke’s Law:
- Hydrogen (H) vs. Deuterium (D): O-H stretching in H₂O occurs around 3400 cm⁻¹, while O-D stretching in D₂O appears near 2500 cm⁻¹.
- Carbon-12 (¹²C) vs. Carbon-13 (¹³C): Carbon isotope substitution causes minor shifts in vibrational frequencies but is useful in isotopic labeling studies.
8. Conjugation and Resonance
Conjugation and resonance affect electron distribution in molecules, modifying bond strengths and altering vibrational frequencies.
- Conjugated systems (C=C-C=C, C=O in amides, esters, and carboxylic acids) lower vibrational frequencies due to electron delocalization, weakening bonds.
- The carbonyl stretching frequency in aldehydes and ketones (~1720 cm⁻¹) shifts to lower values in esters (~1735 cm⁻¹) and amides (~1650 cm⁻¹) due to resonance stabilization.
- Aromatic compounds exhibit characteristic stretching and bending frequencies due to resonance stabilization.
9. Steric Effects
Steric hindrance refers to the influence of bulky groups near a vibrating bond. These steric effects can restrict molecular flexibility, alter bond lengths, and modify force constants, affecting vibrational frequencies.
- Bulky substituents restrict bond rotation, altering vibrational characteristics.
- Intramolecular steric interactions can cause unusual peak shifts in IR spectra.
10. Temperature and Pressure Effects
Vibrational frequencies can change with temperature and pressure, albeit usually by small amounts.
- Increasing temperature increases molecular motion, sometimes reducing hydrogen bonding, leading to higher vibrational frequencies.
- High pressure can compress molecular bonds, altering force constants and causing frequency shifts.
- Some functional groups show broadening of absorption peaks at elevated temperatures due to increased anharmonicity of vibrations.
Conclusion
The vibrational frequencies observed in IR spectroscopy are influenced by multiple factors, including atomic mass, bond strength, bond length, dipole moment changes, molecular environment, isotopic substitution, conjugation, steric effects, and external conditions such as temperature and pressure. Understanding these factors is crucial for accurate spectral interpretation and molecular characterization. By considering these influences, scientists can use IR spectroscopy to identify functional groups, study molecular interactions, and elucidate chemical structures with high precision.
Visit to: Pharmacareerinsider.com