Modes of Molecular Vibrations
Molecular Vibrations
Modes of Molecular Vibrations: Molecules are dynamic entities, constantly in motion due to the vibrational activity of their constituent atoms. This vibrational activity arises because chemical bonds behave like springs that can stretch, bend, and twist when subjected to external forces. The energy associated with these vibrations falls within the infrared (IR) region of the electromagnetic spectrum, allowing IR spectroscopy to be a powerful analytical tool for studying molecular structures.
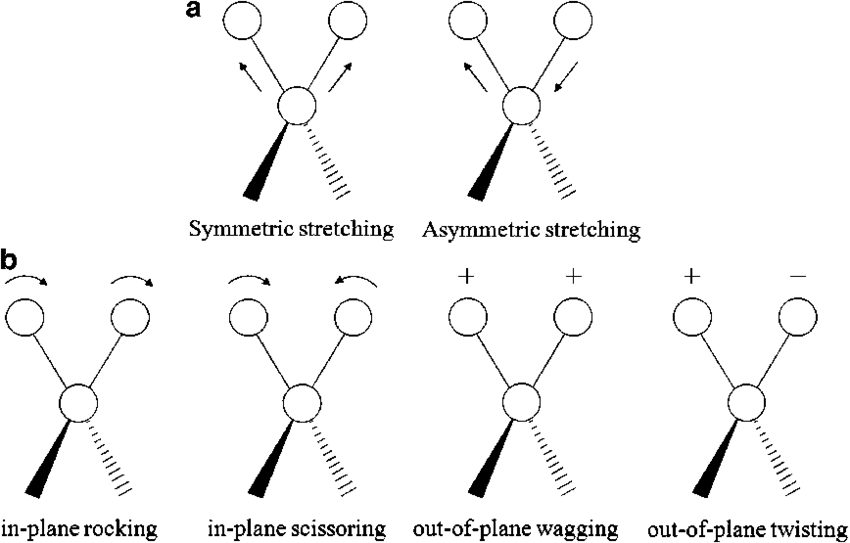
Molecular vibrations can be broadly classified into two main types: stretching and bending. When a molecule absorbs IR radiation, it causes a change in the dipole moment, leading to transitions between vibrational energy levels. This absorption results in characteristic peaks in an IR spectrum, which are used to identify molecular structures, functional groups, and bonding environments.
Modes of Molecular Vibrations
In IR spectroscopy, molecular vibrations are categorized based on the type of motion involved:
1. Stretching Vibrations
Stretching vibrations involve the movement of atoms along the bond axis. They can be further classified into:
- Symmetric Stretching: In this mode, two or more atoms move in unison, symmetrically away from or towards the central atom. This means that both bonds elongate or contract simultaneously at the same rate.
Example: In carbon dioxide (CO₂), the two C=O bonds can stretch in phase, resulting in symmetric stretching.
- Asymmetric Stretching: In this mode, two or more atoms move unequally, leading to an asymmetric motion where one bond elongates while another contracts.
Example: In CO₂, one C=O bond may stretch while the other contracts, producing an asymmetric stretching vibration.
2. Bending Vibrations
Bending vibrations involve the change in the angle between two bonds. These are generally of lower energy compared to stretching vibrations and can be classified into:
- Scissoring: Two adjacent atoms move towards or away from each other in a manner similar to a pair of scissors opening and closing.
Example: The C-H bonds in methylene (-CH₂-) groups exhibit scissoring vibrations.
- Rocking: The movement of atoms or groups of atoms in a back-and-forth manner while maintaining the bond angle. This motion resembles the rocking of a chair.
Example: Rocking motions are commonly observed in long-chain hydrocarbons and benzene derivatives.
- Twisting: Atoms or groups of atoms rotate around a bond axis in opposite directions, creating a twisting effect.
Example: Twisting is observed in molecules with complex ring structures like cycloalkanes.
- Wagging: The movement of atoms or groups of atoms in a sideways motion, resembling the wagging of a dog’s tail.
Example: Found in amine (-NH₂) and methylene (-CH₂-) groups.
- Out-of-Plane Bending: Involves the movement of atoms or groups of atoms perpendicular to the plane of the molecule.
Example: This is commonly seen in aromatic rings, where hydrogen atoms undergo out-of-plane bending vibrations.
Factors Affecting Vibrational Frequencies
Several factors influence the vibrational frequencies observed in IR spectroscopy:
- Bond Strength: Stronger bonds (such as C≡C or C≡N) vibrate at higher frequencies compared to weaker bonds (such as C-C or C-N).
- Atomic Mass: Lighter atoms vibrate at higher frequencies compared to heavier atoms. For example, C-H vibrations occur at higher frequencies than C-Cl vibrations.
- Molecular Environment: Hydrogen bonding, conjugation, and electronic effects can shift vibrational frequencies.
- Resonance Effects: Delocalization of electrons in conjugated systems can lower the vibrational frequencies of bonds.
- Hybridization: The vibrational frequency of a bond depends on the hybridization state of the atoms involved. For instance, sp-hybridized C-H bonds vibrate at higher frequencies than sp² or sp³ hybridized C-H bonds.
Selection Rules in IR Spectroscopy
Not all vibrational modes are IR active. The ability of a molecular vibration to absorb IR radiation is governed by selection rules:
- A vibration is IR active if it results in a change in the dipole moment (∆μ ≠ 0). The greater the change in dipole moment, the stronger the IR absorption.
- Vibrations that do not cause a dipole moment change (∆μ = 0) are IR inactive and do not appear in the IR spectrum. Such vibrations can be detected using Raman spectroscopy instead.
Application of Molecular Vibrations in IR Spectroscopy
IR spectroscopy is widely used for the identification and characterization of molecules based on their vibrational spectra. Some important applications include:
- Functional Group Identification: Each functional group (e.g., -OH, -C=O, -NH₂) has a characteristic vibrational frequency range, allowing their identification in unknown compounds.
- Structure Determination: By analyzing vibrational modes, chemists can determine molecular geometry and bonding.
- Quantitative Analysis: The intensity of absorption bands in an IR spectrum can be used to determine the concentration of a specific functional group in a sample.
- Polymer and Material Science: IR spectroscopy is essential in studying polymer structures, degree of crystallinity, and degradation.
- Pharmaceutical Analysis: Used for quality control, detecting impurities, and verifying molecular structures in drugs and formulations.
Characteristic IR Absorption Ranges
Below are some typical absorption frequency ranges for common functional groups:
Functional Group | Vibration Type | Wavenumber Range (cm⁻¹) |
O-H (alcohols) | Stretching | 3200-3600 |
C-H (alkanes) | Stretching | 2800-3000 |
C=O (carbonyl) | Stretching | 1650-1750 |
N-H (amines) | Stretching | 3300-3500 |
C≡C (alkynes) | Stretching | 2100-2260 |
C≡N (nitriles) | Stretching | 2210-2260 |
Conclusion
Infrared (IR) spectroscopy provides a detailed understanding of molecular vibrations, helping researchers identify molecular structures with high precision. The classification of vibrations into stretching and bending modes enables the interpretation of characteristic absorption bands in an IR spectrum. By analyzing these vibrational modes, scientists can determine functional groups, study chemical interactions, and verify molecular identities in various fields such as pharmaceuticals, polymers, and materials science. The application of IR spectroscopy in routine analytical and research laboratories makes it an indispensable tool in modern chemistry and material characterization.