Muscle contraction is a complex physiological process that involves the activation of muscle fibers, resulting in the shortening of the muscle and the generation of force. It plays a fundamental role in various physiological functions, including movement, posture maintenance, and the regulation of many bodily processes. Here’s a detailed note on the physiology of muscle contraction:
1. Excitation-Contraction Coupling:
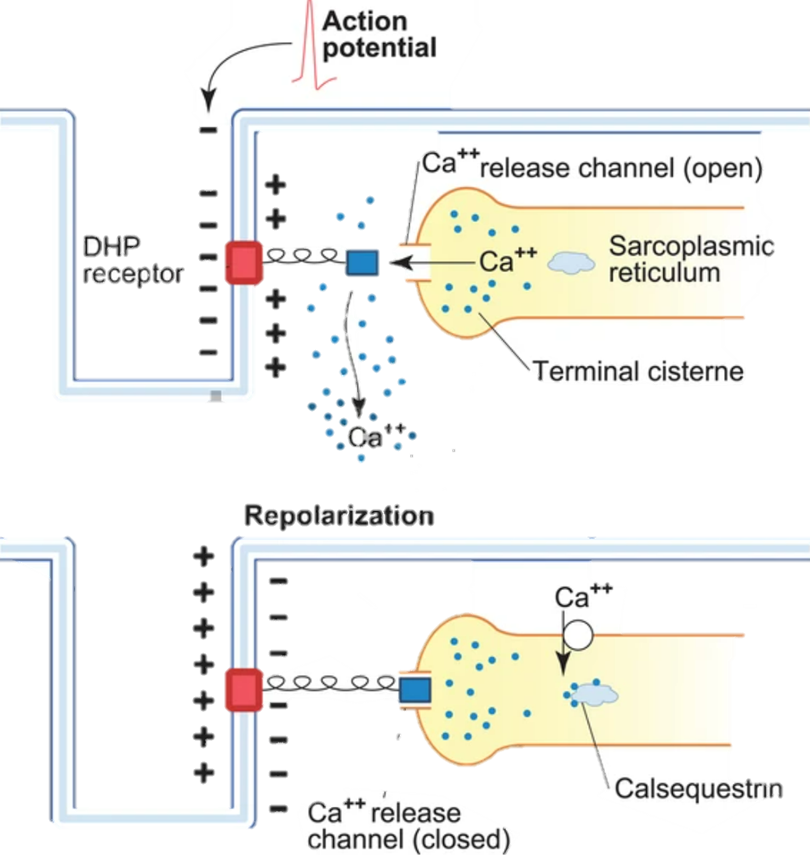
The process of muscle contraction begins with a neural signal. When a motor neuron sends an action potential (nerve impulse) to a muscle fiber, it reaches the neuromuscular junction—the point of contact between the motor neuron and the muscle fiber.
At the neuromuscular junction, the action potential triggers the release of the neurotransmitter acetylcholine (ACh) from vesicles in the motor neuron’s axon terminal. ACh binds to receptors on the muscle fiber’s sarcolemma (cell membrane), leading to depolarization.
The depolarization of the sarcolemma spreads along the muscle fiber’s T-tubules (transverse tubules), which are invaginations of the sarcolemma, and into the sarcoplasmic reticulum (SR), a specialized organelle that stores calcium ions (Ca2+).
In response to the depolarization, the SR releases stored Ca2+ ions into the sarcoplasm (cytoplasm of the muscle fiber).
2. Sliding Filament Theory:
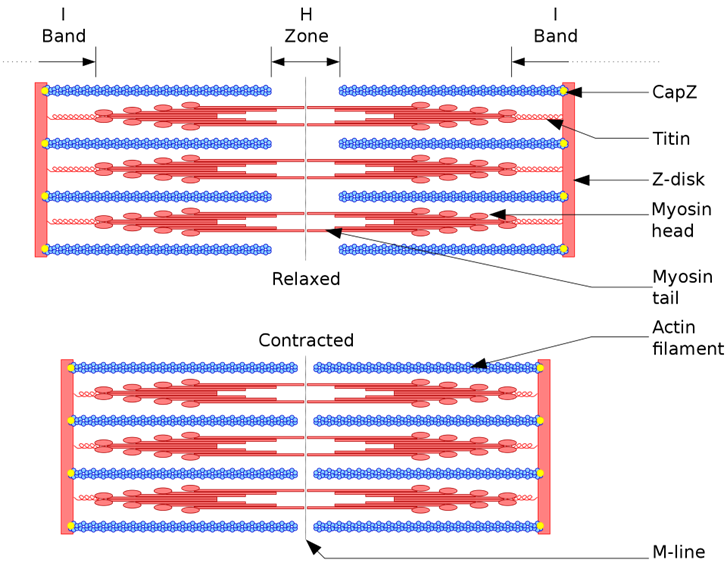
The sliding filament theory primarily drives muscle contraction, describing how myofilaments (protein filaments) within muscle fibers interact to generate force.
In a relaxed muscle, tropomyosin and troponin block myosin heads (thick filaments) from binding to actin (thin filaments). When calcium ions are released into the sarcoplasm, they bind to troponin, causing it to change shape and move tropomyosin away from the myosin-binding sites on actin.
Myosin heads then bind to actin, forming cross-bridges. Energy from the hydrolysis of ATP (adenosine triphosphate) pivots the myosin heads, causing the thin filaments to slide past the thick filaments.
As long as calcium ions are present, the cross-bridges repeatedly cycle through attachment, power stroke, and detachment, causing the sarcomeres (contractile units of muscle) to shorten.
3. Muscle Contraction and Force Generation:
The number of sarcomeres determines the force of muscle contraction in a muscle fiber that is actively contracting. The greater the number of cross-bridges formed between actin and myosin, the greater the force generated.
The frequency and strength of action potentials in motor neurons also play a role in controlling the force of contraction. Recruitment of more motor units (groups of muscle fibers innervated by a single motor neuron) leads to more muscular contractions.
4. Muscle Relaxation:
Muscle relaxation occurs when the stimulation of the motor neuron ceases. The action potential stops, and acetylcholine is broken down by acetylcholinesterase, preventing further depolarization.
Calcium ions are actively pumped back into the SR, reducing their concentration in the sarcoplasm. As calcium ions detach from troponin, tropomyosin returns to its blocking position, covering the myosin-binding sites on actin.
As the cross-bridges detach and the myosin heads return to their original positions, the sarcomeres lengthen and the muscle relaxes.
5. Energy for Muscle Contraction:
Muscle contraction requires a continuous supply of ATP for the energy needed to power the myosin heads. ATP is obtained through various metabolic pathways, including aerobic respiration, anaerobic glycolysis, and the creatine phosphate system.
6. Muscle fatigue:
Muscle fatigue occurs when a muscle cannot sustain or generate force due to prolonged or strenuous activity. It can result from factors such as ATP depletion, lactic acid accumulation, and the buildup of metabolic waste products. Understanding muscle contraction physiology is essential for various fields, including sports science, physical therapy, and medicine. It enables researchers and practitioners to develop strategies for enhancing muscle performance, preventing muscle injuries, and treating muscle-related disorders.