Quenchers
Quenchers: Quenching is a fundamental process in fluorescence spectroscopy, where the intensity of fluorescence emission is reduced due to interactions with specific molecules known as quenchers. Fluorescence is a widely used analytical technique that relies on the ability of certain molecules, known as fluorophores, to absorb light at a specific wavelength and subsequently emit light at a longer wavelength. However, under certain conditions, this fluorescence can be diminished or completely suppressed, a phenomenon known as fluorescence quenching. Quenching can be either desirable or undesirable, depending on the context in which fluorescence is being used. In analytical chemistry, quenching can provide valuable information about molecular interactions, binding affinities, and environmental factors affecting fluorophores.
Suggested post: Click here
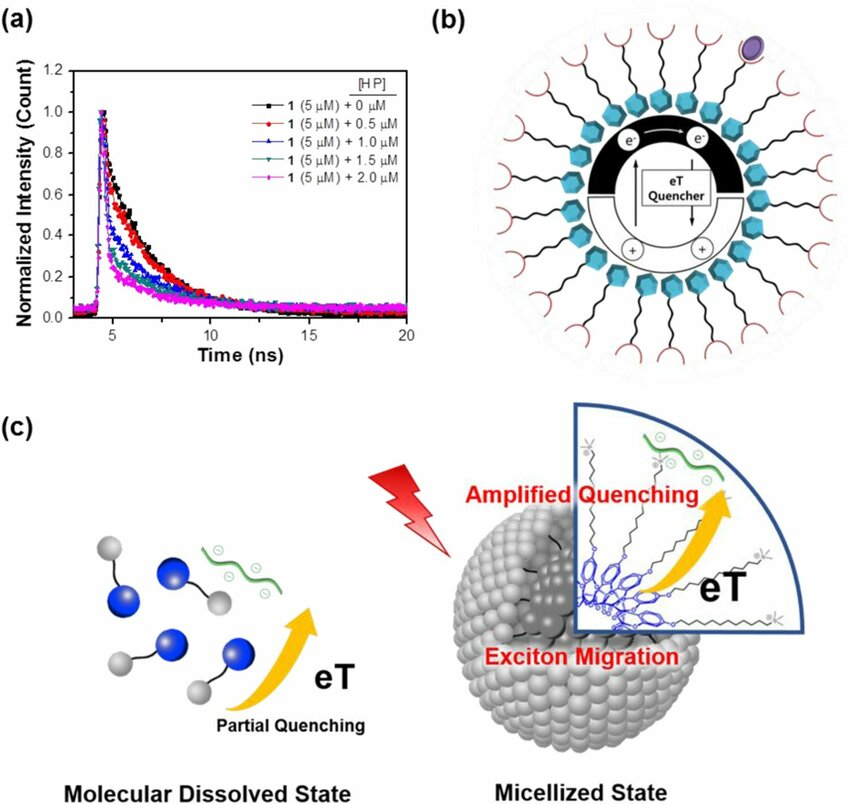
The process of quenching plays a crucial role in various scientific fields, including biochemical assays, environmental monitoring, pharmaceutical analysis, and fluorescence-based imaging techniques. Quenching can occur through different mechanisms, such as collisional quenching, static quenching, energy transfer, and charge transfer. Understanding these mechanisms is essential for optimizing fluorescence-based experiments, improving sensitivity, and preventing errors in fluorescence analysis.
2. Mechanisms of Quenching
Fluorescence quenching is not a singular process but rather a set of distinct phenomena that lead to the reduction in fluorescence intensity. Several different mechanisms contribute to fluorescence quenching, each with unique characteristics and implications. These include:
2.1 Collisional (Dynamic) Quenching
Collisional quenching, also known as dynamic quenching, occurs when a quencher molecule comes into transient contact with a fluorophore while it is in its excited state. During this interaction, the energy of the excited fluorophore is transferred to the quencher molecule, leading to non-radiative energy dissipation and a reduction in fluorescence intensity. This type of quenching is highly dependent on diffusion and is therefore influenced by factors such as temperature, solvent viscosity, and the nature of the quencher.
Collisional quenching is mathematically described by the Stern-Volmer equation:
where:
- represents the fluorescence intensity in the absence of a quencher,
- represents the fluorescence intensity in the presence of a quencher,
- is the Stern-Volmer quenching constant, which reflects the efficiency of the quenching interaction,
- represents the concentration of the quencher.
Common collisional quenchers include molecular oxygen (O₂), halide ions (I⁻, Br⁻, Cl⁻), and small organic molecules such as acrylamide. The effectiveness of collisional quenching depends on the frequency and strength of encounters between the fluorophore and the quencher.
2.2 Static Quenching
Unlike collisional quenching, static quenching does not involve transient molecular interactions in the excited state. Instead, static quenching occurs when the fluorophore forms a stable, non-fluorescent complex with the quencher in the ground state before excitation occurs. This complex prevents the fluorophore from fluorescing when excited by light. Static quenching is particularly relevant in cases where fluorophores and quenchers have a strong binding affinity, leading to significant fluorescence suppression.
The Stern-Volmer equation for static quenching is modified as follows:
where is the static quenching constant, which differs from the Stern-Volmer quenching constant for dynamic quenching.
Static quenching is often confirmed using absorption spectroscopy. If complex formation occurs, characteristic changes in the absorption spectrum of the fluorophore can be observed, indicating the presence of static quenching.
2.3 Förster Resonance Energy Transfer (FRET)
Förster Resonance Energy Transfer (FRET) is a specialized quenching mechanism that occurs when an excited fluorophore (the donor) transfers energy to a nearby acceptor molecule (the quencher) without emitting a photon. This transfer occurs via dipole-dipole interactions and is highly dependent on the distance between the donor and acceptor, typically in the range of 1-10 nm.
The efficiency of FRET is influenced by several factors, including:
- The spectral overlap between the emission spectrum of the donor and the absorption spectrum of the acceptor.
- The distance between the donor and acceptor, with efficiency decreasing rapidly as distance increases.
- The relative orientation of the dipole moments of the donor and acceptor molecules.
FRET is widely used in molecular biology, where it is employed to study biomolecular interactions, protein conformational changes, and signal transduction pathways. The ability to monitor real-time interactions at the nanoscale makes FRET a valuable tool in fluorescence-based biosensing.
2.4 Charge Transfer Quenching
Charge transfer quenching occurs when an electron is transferred between the fluorophore and the quencher, leading to non-radiative decay of the excited-state fluorophore. This type of quenching is commonly observed in organic molecules with strong electron donor-acceptor interactions. Charge transfer quenching is significant in photochemistry and electrochemical fluorescence applications, where electron transfer dynamics are crucial for understanding reaction mechanisms.
2.5 Excited-State Reactions
Some fluorescence quenching occurs due to chemical reactions that take place when the fluorophore is in its excited state. These reactions, such as photo-induced electron transfer (PET), alter the chemical structure of the fluorophore and prevent fluorescence emission. This type of quenching is particularly relevant in photochemical reactions and fluorescence-based sensing applications.
3. Common Quenchers and Their Applications
There are many known quenchers, each with specific applications in fluorescence studies. These quenchers include:
3.1 Molecular Oxygen (O₂)
Molecular oxygen is one of the most common and effective quenchers in fluorescence studies. It primarily acts via collisional quenching, where its interaction with an excited fluorophore leads to energy dissipation. Oxygen quenching plays a significant role in photodynamic therapy and oxygen-sensing applications.
3.2 Halide Ions (I⁻, Br⁻, Cl⁻)
Halide ions, such as iodide (I⁻), bromide (Br⁻), and chloride (Cl⁻), are well-known quenchers that operate through collisional quenching. The efficiency of halide quenching follows the order: I⁻ > Br⁻ > Cl⁻, due to differences in their electron cloud interactions with fluorophores.
3.3 Acrylamide
Acrylamide is frequently used in fluorescence quenching studies, particularly in protein research. It is commonly used to probe the fluorescence quenching of tryptophan residues in proteins, helping to elucidate protein structure and dynamics.
3.4 Synthetic Quenchers (Dabcyl, Black Hole Quenchers)
Synthetic dyes, such as Dabcyl and Black Hole Quenchers (BHQ), are frequently used in fluorescence resonance energy transfer (FRET)-based molecular probes. These quenchers are commonly used in nucleic acid hybridization assays and real-time polymerase chain reaction (PCR) techniques.
4. Applications of Quenching in Scientific Research
Fluorescence quenching has a wide range of applications, including:
4.1 Fluorescence-Based Biosensors
Biosensors use quenching mechanisms to detect biomolecules, toxins, and environmental pollutants with high sensitivity.
4.2 Drug Discovery
Quenching studies help analyze drug-receptor interactions, enzyme inhibition, and protein-ligand binding.
4.3 Medical Imaging
Fluorescence quenching techniques are used in fluorescence microscopy, PET scans, and near-infrared imaging for disease diagnosis.
Conclusion
Fluorescence quenching is a fundamental process with diverse applications in analytical chemistry, biology, and medicine. Understanding quenching mechanisms enhances the accuracy of fluorescence-based experiments and enables the development of innovative sensing and imaging technologies.