Introduction to Spectrofluorimetry
Spectrofluorimetry is an advanced analytical technique employed to measure the fluorescence emitted by a substance when it is excited by a specific wavelength of light. This method plays a crucial role in various scientific disciplines such as biochemistry, pharmacology, environmental science, and forensic analysis, where it is used to identify, quantify, and characterize fluorescent molecules. The principle underlying spectrofluorimetry is fluorescence, a form of photoluminescence in which a molecule absorbs light energy and re-emits it at a longer wavelength.
Suggested Post: Click here
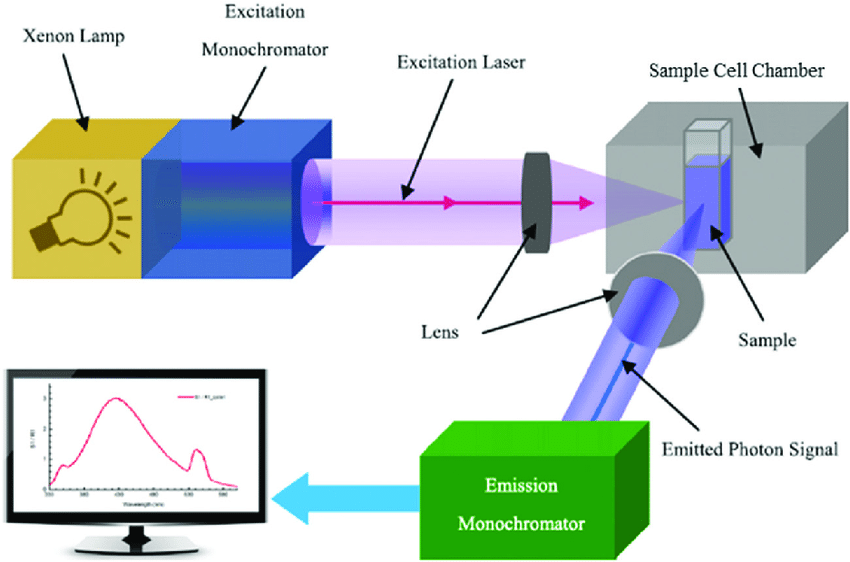
Theory of Fluorescence
Fluorescence is a physical phenomenon wherein certain molecules absorb photons of light at one wavelength and subsequently emit photons at a longer wavelength due to energy loss. The process of fluorescence occurs in distinct phases:
- Excitation: When a molecule absorbs a photon, an electron within the molecule transitions from the ground state (S0) to an excited singlet state (S1, S2, etc.). The energy of the absorbed photon must correspond to the energy difference between these electronic states.
- Relaxation (Internal Conversion and Vibrational Relaxation): Before fluorescence emission, the excited molecule undergoes non-radiative relaxation processes, including internal conversion and vibrational relaxation, which result in energy dissipation as heat, bringing the molecule to the lowest vibrational level of the first excited state (S1).
- Emission: The molecule eventually returns to the ground state (S0) by emitting a photon, which is observed as fluorescence. The emitted photon possesses less energy than the absorbed photon, leading to a shift in the emitted wavelength.
Jablonski Diagram
The fluorescence mechanism can be visualized using a Jablonski diagram, which illustrates the electronic states of a molecule and the transitions between them:
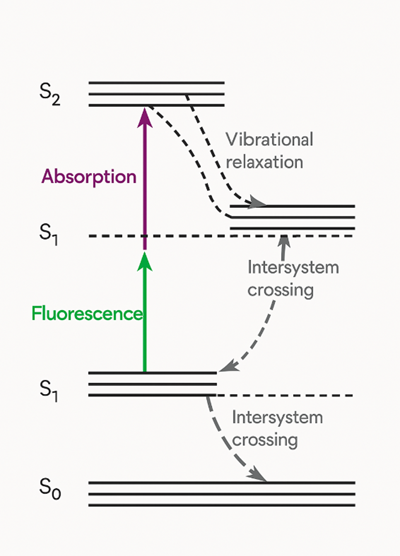
- The diagram includes the ground electronic state (S0) and multiple excited singlet states (S1, S2, etc.).
- The excitation process moves electrons to higher energy states.
- Non-radiative relaxation processes such as internal conversion and vibrational relaxation cause energy loss.
- Fluorescence emission occurs when the electron transitions back from S1 to S0, releasing a photon of lower energy.
Stokes Shift
Stokes shift refers to the spectral difference between the absorbed and emitted light. Due to energy loss through vibrational relaxation before emission, the fluorescence emission occurs at a longer wavelength than the excitation wavelength. This shift is critical in fluorescence spectroscopy as it facilitates the differentiation between excitation and emission spectra, thereby enhancing detection sensitivity.
Fluorescence Lifetime
Fluorescence lifetime represents the average duration a molecule remains in the excited state before returning to the ground state via photon emission. Typically measured in nanoseconds, fluorescence lifetime is influenced by the molecular environment, electronic interactions, and quenching effects. This parameter provides essential insights into molecular interactions, energy transfer, and dynamic processes within complex biological and chemical systems.
Quantum Yield
Quantum yield (Φ) is a measure of fluorescence efficiency, defined as the ratio of emitted photons to absorbed photons: Φ = (Number of photons emitted) / (Number of photons absorbed)
A high quantum yield indicates that a greater proportion of absorbed photons result in fluorescence emission, while a low quantum yield suggests alternative non-radiative energy dissipation mechanisms.
Factors Affecting Fluorescence
Numerous factors influence the fluorescence characteristics of a molecule:
- Molecular Structure: Aromatic compounds with rigid conjugated structures often exhibit strong fluorescence due to restricted molecular motion.
- Solvent Effects: The polarity and refractive index of solvents can alter excited-state properties, influencing fluorescence intensity and emission wavelength.
- pH and Ionic Strength: The protonation state of fluorophores can change in different pH environments, modifying fluorescence behavior.
- Quenching Mechanisms: Fluorescence intensity can be reduced due to interactions with quenchers such as oxygen, heavy metals, or specific chemical groups, through static or dynamic quenching mechanisms.
- Temperature Variations: Increased temperature enhances non-radiative relaxation pathways, leading to decreased fluorescence intensity.
Applications of Spectrofluorimetry
Spectrofluorimetry has diverse applications across scientific and industrial fields:
- Biochemical and Medical Research: Applied in the study of biomolecules, protein folding, enzyme kinetics, and cellular imaging.
- Pharmaceutical Analysis: Used in drug formulation studies, stability assessments, and detection of pharmaceutical contaminants.
- Environmental Monitoring: Enables the detection and quantification of pollutants, including heavy metals, polycyclic aromatic hydrocarbons, and pesticides.
- Forensic Science: Helps in the identification of drugs, biological stains, and counterfeit materials.
- Food Safety and Quality Control: Used to monitor food additives, vitamins, and spoilage markers in consumables.
Conclusion
Spectrofluorimetry is a highly sensitive and versatile analytical technique grounded in the principles of fluorescence. The study of fluorescence, including excitation, relaxation, and emission mechanisms, allows researchers to exploit fluorescence spectroscopy for a wide range of applications. By understanding factors such as solvent effects, molecular interactions, and quenching mechanisms, fluorescence measurements can be optimized to provide precise and meaningful analytical data. As technological advancements continue, spectrofluorimetry remains an indispensable tool for modern scientific research and industrial applications.
Well explain.
thank you Pharma team.